- What are biomaterials?
- What are the benefits of biomaterial technologies and how are they being used?
- What are NIBIB-funded researchers developing in the area of biomaterials to improve medical care?
What are biomaterials?
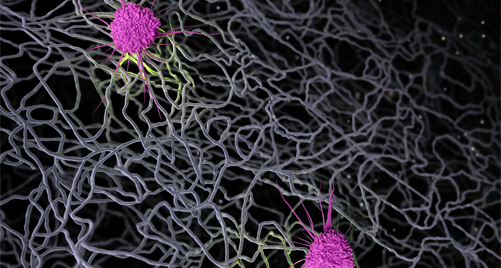
Biomaterials are all around us and, sometimes, within us too. In medical devices as simplistic as dental fillings to as complex as spinal cord stimulators, these materials interact with the human body to fulfill medical or cosmetic needs.
People have used biomaterials from natural sources dating back to ancient times, with some of the earliest examples being prosthetics carved from wood and animal tendons used as sutures. Since those humble beginnings, humans have developed means of producing a variety of synthetic polymers, metals, and ceramics for healthcare applications.
Now, we reengineer both natural and artificial materials into molded or machined parts, coatings, fibers, films, foams, fabrics and more for use in biomedical technologies, such as heart valves, knee joint replacements, dental implants, or contact lenses. Biomaterials can also provide a structural framework in tissue-engineered products for tissue engineering that aim to restore, maintain, or improve damaged tissues or organs.
The field of biomaterials spans a wide variety of biomedical applications, and thus, is highly interdisciplinary by nature, incorporating aspects of medicine, biology, chemistry, materials science, and pharmacology.
What are the benefits of biomaterial technologies and how are they being used?
They can enhance biocompatibility. When foreign objects enter the body, they are usually met with hostility, as the immune system first attempts to degrade them and eventually encases them in scar tissue. This usually happens for good reason, but when the foreign body is a medical device, this process, known as the foreign body response (FBR), can hinder device function, cause pain, and lead to additional surgeries.
To minimize FBR, many medical devices employ corrosion-resistant, biologically inert materials, such as titanium and silicone rubber. Another strategy is to use biodegradable materials that break down before FBR becomes a chronic issue. Materials can also be designed to mimic aspects of the native scaffolding that cells grow within, called the extracellular matrix (ECM).
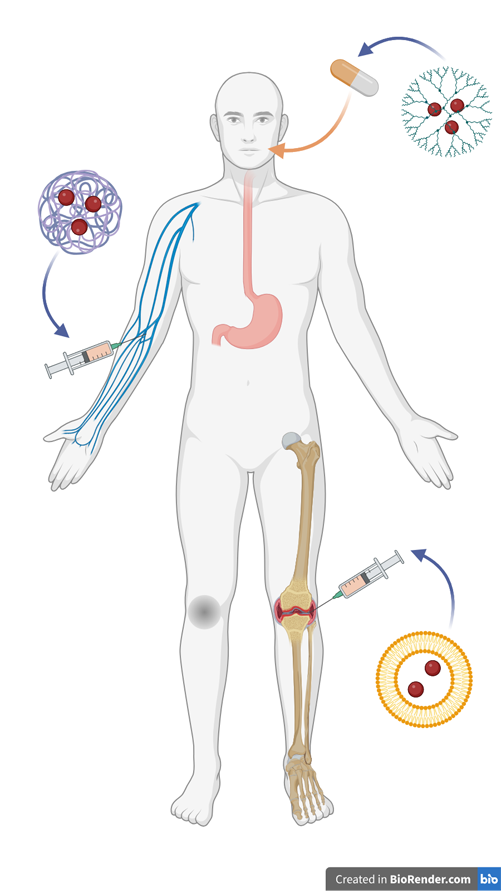
By matching the stiffness, structure, or chemical composition of native ECM, materials can convey friendly signals that not only reduce FBR but encourage healing and tissue integration. One common example is the use of hydroxyapatite – an abundant mineral in bone – for orthopedic implant coatings, which encourages tissue ingrowth and increases device longevity.
They can restore or replace tissue function. Biomaterials confer important properties to medical devices that allow them to treat diseased or injured tissue. In load-bearing technologies, such as artificial hip joints, metal alloys and ceramics that are strong enough to support surrounding tissue and wear-resistant enough to withstand repetitive motion are generally implemented.
Mechanical properties are just as critical when devices engage with soft tissues. For example, artificial blood vessels and vascular stents made of adequately flexible polymers and metal alloys can support pulsatile blood flow in place of or together with deficient vessels. Materials may fulfill a need due to other characteristics, such as optical properties. To restore visual acuity, intraocular or contact lenses made of silicone or some other polymer must be sufficiently transparent and refract light at the appropriate angle.
They can deliver therapeutics. Putting medication into the bloodstream has been a tried-and-true method of treatment for thousands of years. A consistent risk that comes with this approach, however, is the possibility of off-target effects. When therapeutics are administered into the circulatory system they may end up in organs and tissues that are not the intended target, sometimes to deleterious effect. Some can be administered locally through alternative routes, such as topical medications. But others must enter the blood. Even then, some may degrade before reaching their targets.
Biomaterials, such as nanoparticles made of synthetic or lipid polymers, can encase therapeutics to help protect them in the bloodstream. These carriers can be modified to be selectively taken up by certain cell populations as well. Medical devices, from tissue scaffolds to vascular stents, can be coated or loaded with anti-inflammatory or anti-fibrotic therapeutics. These can be released in a controlled manner, through the biodegradation of the material holding the therapeutic, or in response to a specific biological stimulus, such as heat or acidity.
What are NIBIB-funded researchers developing in the area of biomaterials to improve medical care?
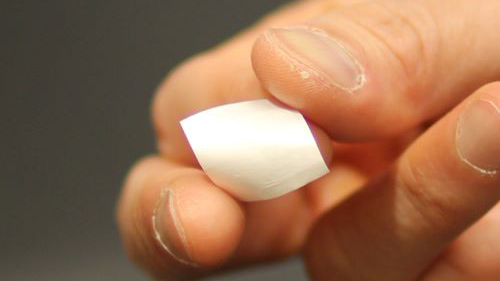
Recharging cartilage after knee damage
Osteoarthritis is a painful joint condition affecting millions worldwide, yet current treatment options are limited. Seeking an alternative, researchers developed a biodegradable polymer film that, when subjected to pressure, produces cartilage-regenerating electrical current. In a model of osteoarthritis in rabbits, the team found that animals treated with the films and a daily exercise routine regenerated new tissue that closely mimicked native cartilage.
New insight into the immune response forges a path toward improved medical implants
Why do some biomaterials elicit harmful immune reactions while others stimulate reparative responses? To find out, NIBIB researchers studied the differences in the immune response to both synthetic and naturally derived materials in an animal model of muscle injury. They uncovered key driving factors for implant-induced regeneration and damage – findings that could inform the design of new medical devices that steer the immune system toward regenerative processes.