Preclinical ultrasound method could reshape surgical procedures, making them safer and less invasive
What if a clinician could 3D print something through your skin, constructing an implant or replacement organ underneath layers of tissue? The world of medicine would be transformed: a host of surgical procedures, which come with a variety of risks, could be performed without ever lifting a scalpel.
A collaborative, NIH-funded team is working to make this Star Trek-like fiction a reality. Their work, published today in Science, outlines a method to print biocompatible structures through thick, multi-layered tissues. The principle? Focused ultrasound, combined with a novel ultrasound-sensitive ink.
“Focused ultrasound has been used for decades to treat a wide variety of conditions, underscoring its safety and utility as a clinical tool,” said Randy King, Ph.D., a program director in the Division of Applied Science & Technology at NIBIB. “This potential new application, built on years of technology advancements, could set the stage for something previously thought impossible: through-tissue 3D ultrasound printing.”
Using ultrasound principles for 3D printing
Some 3D printing techniques use light—a form of energy—to trigger the solidification of an “ink,” which is often composed of liquid substance, like resin or plastic. Light, however, doesn’t travel well through skin and organs, getting scattered as it passes through them. In order to successfully “print” through biological tissues, a different energy source would be needed.
Enter ultrasound. Compared with light, ultrasound waves can travel much further through tissues, sending energy well below the surface. By delivering an ink that could be solidified through interactions with ultrasound waves, a clinician could potentially build biologically relevant structures inside of the human body, all without making an incision.
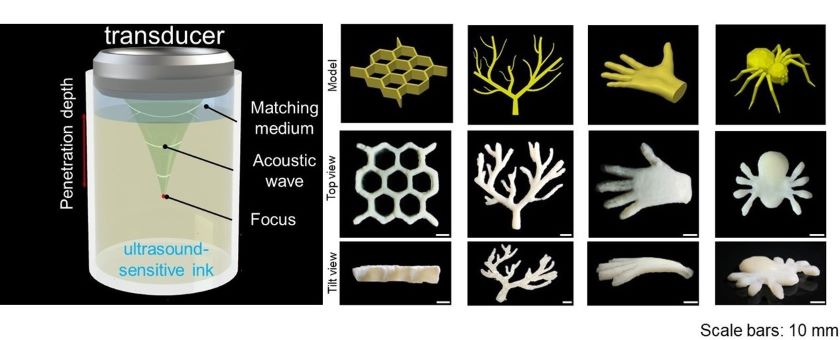
“Our technology is based on the sono-thermal effect, which describes the increase in temperature that occurs due to the absorption of ultrasound waves,” explained senior study author Junjie Yao, Ph.D., an associate professor of biomedical engineering at Duke University. “If we can precisely control the temperature increase by focusing the ultrasound waves, we can guide the solidification of the injected ink, even through layers of tissue.”
The ink is made using four components: a compound that helps to absorb the ultrasound waves, a microparticle that helps to control viscosity, a polymer that provides structure, and a salt that absorbs heat to trigger solidification. “The individual components of the bioink are not new—in fact, they have been used for many years in biomaterials applications,” said senior study author Y. Shrike Zhang, Ph.D., associate professor and associate bioengineer at Harvard Medical School and Brigham and Women’s Hospital, respectively. “The key was the combination of these ingredients, in the appropriate ratios, which allowed us to create an ink that can rapidly solidify upon interactions with ultrasound waves without getting dispersed.”
From principle to proof of concept
To start, the researchers wanted to see if they could create 3D shapes using their ultrasound technique. They suspended a focused ultrasound transducer over a chamber filled with their novel ink. Between the transducer and the ink was a “matching medium,” a substance used in most ultrasound methods that ensures efficient transmission of ultrasound waves. Using a computer program to precisely control the intricate 3D movements of the ultrasound transducer, the researchers were able to create a variety of different structures at varying depths inside of the ink chamber. These structures had a range of sizes and geometrical intricacies and included objects such as multi-layer honeycomb, a branched vascular network, and complex models that resembled a hand or spider.
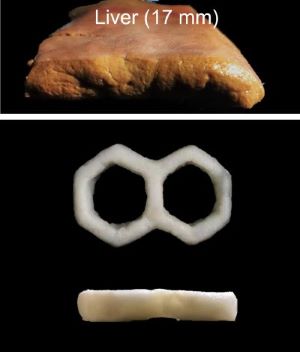
Next, the researchers wanted to determine if their technique could be used to print through biological tissues. They placed pig tissues that ranged in thickness (up to 17 millimeters) on top of the chamber filled with ink. With the transducer positioned above, the researchers steered ultrasound waves through the tissue and into the chamber below. They successfully printed a variety of different structures through several different kinds of tissues, including a pig liver and a pig tissue phantom that was comprised of multiple layers (such as skin, fat, and muscle).
To mimic a real-world procedure, the researchers performed a mock surgery using an ex vivo goat heart. With a catheter, the researchers injected their ultrasound-sensitive ink into the left atrial appendage, a sac-like structure in the heart where blood clots can develop. Through the goat heart wall, the researchers used ultrasound waves to solidify the ink, effectively sealing off this area of the heart. This procedure, called left atrial appendage closure, can require open-heart surgery and is sometimes performed on patients with atrial fibrillation with increased risk of stroke.
These initial experiments demonstrate that this proof-of-concept printing method has the potential to turn highly invasive surgical procedures into safer, less invasive ones. “Our technique could provide an unconventional avenue towards truly minimally invasive medicine which seemed nearly impossible before,” said Yao.
Looking ahead
This focused ultrasound printing technique can require high levels of energy, which has the potential to overheat the surrounding tissues. To combat this, the researchers constructed a confocal high-intensity ultrasound printer. This system uses two ultrasound transducers that are aligned in a crossbeam pattern, allowing the two ultrasound wavefronts to overlap. Not only does this design reduce the energy needed from each transducer, it also improves the resolution and speed of the ultrasound printer.
“We will continue to modify our technique to increase its safety and efficiency, looking to adapt our technology for different medical uses,” said Zhang. “While we believe that our method has the potential to enable a variety of minimally invasive procedures, it’s still a prototype technology that will require further optimization before it can be evaluated in humans.”
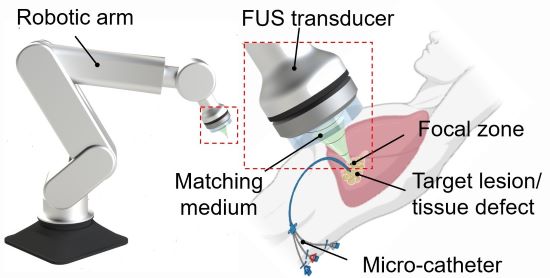
Yao and Zhang—who have known each other since graduate school—have spent the last several years working together on their 3D ultrasound printing technology. “Our multi-year collaboration gave us a chance to create a new technique that combines our unique perspectives: ultrasound imaging and biochemistry,” said Yao. “We could not have created this technology without one another, highlighting the unique possibilities that can stem from interdisciplinary partnerships.”
This research was supported by grants from NIH, including NIBIB (R21EB025270, R21EB027981, R21EB027304, R01EB028143, R21EB027981, and R01EB031629); the National Heart, Lung, and Blood Institute (R01HL153857, R01HL165176, and R01HL166522); the National Institute of Neurological Disorders and Stroke (RF1NS115581 and R01NS111039); and the National Cancer Institute (R01CA282451). Other funding includes grants from the National Science Foundation.
This Science Highlight describes a basic research finding. Basic research increases our understanding of human behavior and biology, which is foundational to advancing new and better ways to prevent, diagnose, and treat disease. Science is an unpredictable and incremental process—each research advance builds on past discoveries, often in unexpected ways. Most clinical advances would not be possible without the knowledge of fundamental basic research.
Study reference: Xiao Kuang et al. Self-enhancing sono-inks enable deep-penetration acoustic volumetric printing. Science 382, 1148-1155 (2023) DOI:10.1126/science.adi1563
The graphics seen here and on the NIBIB website have been adapted from figures found in the Science publication.