Injected neuron-like electronics integrate stably and seamlessly into mouse brain
Standard neural probes to record brain function are eventually seen as foreign and are degraded by attacks from the immune system. Now, researchers funded by NIBIB have designed neuron-like probes that can be implanted and remain viable for long-term use to study and treat the brain. Potential applications include treatments for neurological disorders such as Parkinson’s and Alzheimer’s.
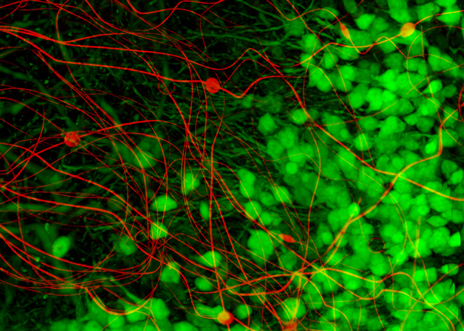
A primary goal of neurological research is to track and map the pattern of neural signals that move through the brain during normal functions and in diseased states. The neural probes currently used in animal studies, and for some procedures in humans, are relatively large and stiff. When these large and stiff devices are inserted into the delicate, sinuous neurons of the brain they can cause damage to the adjacent cells. An inflammatory response builds scar tissue around the probes, greatly reducing the ability to closely monitor these patterns of neural activity.
Now biomaterials scientists at Harvard University, led by Charles Lieber, Ph.D., of the Chemistry and Chemical Biology, Center for Brain Science, and the John A. Paulson School of Engineering and Applied Sciences, have fabricated neural probes that physically mimic brain neurons, evade the immune response, and continue to function for months. The work is described in the February issue of Nature Materials.1
“Designing high resolution neural probes that remain viable in the brain has been a goal of researchers for decades,” explains Michael Wolfson, Ph.D., director of the NIBIB program for Therapeutic Medical Devices. “Dr. Lieber and his group are at the forefront of this endeavor. In this latest work, they have created neural probes that are the size, shape and flexibility of real neurons. These new probes seamlessly integrated and recorded the function of adjacent neurons in mice over relatively long periods of time without inducing the damage and disruption that has hampered this type of work in the past.”
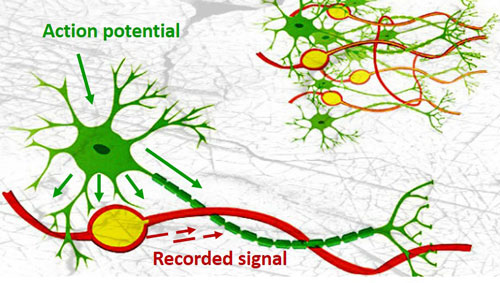
The team built their tiny neuron-mimicking probes using photolithography, the same technique used to fabricate the miniscule and complex circuits in computer chips. The technique allowed the researchers to create neuron-like electronics (NeuE) with a metal center to conduct electricity surrounded by two insulating layers with a total width of a typical neuron, which is about 1/200th the width of a human hair.
Sixteen strands of NeuE were bundled and injected into the hippocampus of mouse brains, which is involved in learning, memory, and aging. The strands unfolded and unobtrusively mingled with brain neurons.
From within a day after implanting the 16 NeuE electronic neurons until 90 days later, when the experiment was ended, the researchers were able to stably pick up brain signals from each individual neuron without losing touch with a single one. This is in sharp contrast to larger, stiffer neural probes, typically five to twenty times the size of the neurons, that initially pick up clear signals from neurons until the immune system builds scar tissue around the probe, blinding researchers to the brain’s activity.
In fact, not only did each of the 16 NeuE electrodes pick up electrical signals from 2-3 distinct neurons on the first day, they also picked up additional signals during the first two weeks, which suggests that additional neurons are connecting to the electrodes. Further studies revealed that the scaffolding created by the web of NeuE electronics was actually attracting newborn neurons that were migrating and interacting with the NeuE electrodes.
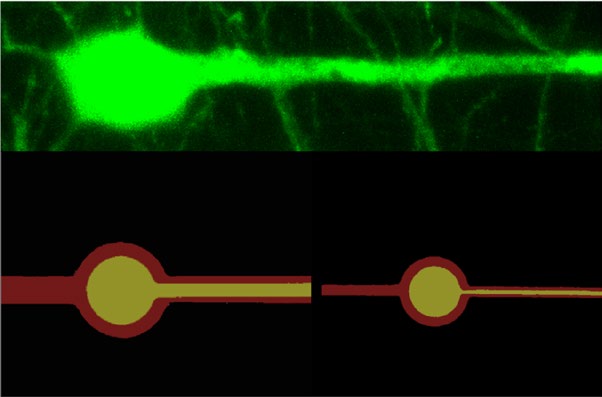
Says Lieber, ”this was a bonus that we did not expect and strongly suggests that webs of NeuE could be implanted in areas where there is degenerative neurologic disease and act to attract new neurons to repopulate the damaged region.”
Lieber stresses that the stability of the system—the ability to unobtrusively integrate in the brain and record brain function for extended periods— is the key. The healthy melding of NeuE with delicate neurons opens potential for long-term implantation and treatment in the brain. He also notes that the brain changes with learning and experience and that such stable long-term implants offer the chance to study this phenomenon as it progresses over time.
Regarding future directions Lieber says, “frankly, this is the most exciting research I have been involved in. My lab members, collaborators and I all have a plethora of ideas for practical applications of this new ‘stealth’ technology. Ideas range from understanding brain development over time to targeting specific neurons involved in diseases like addiction, depression, Parkinson’s and Alzheimer’s to assess disease progression, while simultaneously accelerating recovery with electric stimulation in the damaged area.”
This work is supported by the National Institute on Drug Abuse of the National Institutes of Health (1R21DA043985-01), an NIH Director’s Pioneer Award (1DP1EB025835-01) from the National Institute of Biomedical Imaging and Bioengineering, the Air Force Office of Scientific Research, the Simmons Awards, the American Heart Association Postdoctoral Fellowship, and an NIH Pathway to Independence Award.