Engineered lipid nanoparticles drive durable gene editing in lungs of mice
Gene-editing therapies—techniques that modify DNA to treat or prevent disease—have the potential to transform the field of drug development. By making precise edits to the genome, problematic genes could be modified or eliminated, representing long-lasting therapies for genetic disorders that currently have no treatment.
Few gene-editing therapies currently exist. Last year, the FDA approved a gene-editing therapy for sickle cell disease, a landmark treatment that uses CRISPR to modify blood stem cells. But like most experimental gene-editing treatments, this therapy requires a stem cell transplant, a costly and time-consuming procedure that involves removing stem cells from a patient, modifying them to correct defects causing the disease, and reintroducing them back to the patient. Finding a way to modify a patient’s stem cells in vivo—without having to remove them from the body—could revolutionize the field of genome editing.
Researchers at UT Southwestern are working to bring in vivo gene editing to the fore. Through rational engineering of lipid nanoparticles, this collaborative team developed a way to effectively target specific organs in the body to precisely deliver therapeutic cargo, including gene-editing molecules. Their research demonstrated that a one-time treatment with their nanoparticles resulted in durable gene editing in mouse lungs for nearly two years. Further, their technique showed promise in correcting a mutation present in a currently untreatable form of cystic fibrosis in several models of the disease. The research was recently published in Science.
“There is a real desire to imagine a one-time injection of a medicine that could correct mutations that are causing and driving diseases,” said senior study author Daniel Siegwart, Ph.D., a professor at UT Southwestern Medical Center. “Our preclinical platform illustrates a potential method to achieve long-term gene editing in the lungs, representing a new treatment approach for a variety of genetic respiratory conditions.”
Getting it SORTed
Lipid nanoparticles, a popular drug delivery method thanks to the success of mRNA COVID-19 vaccines, are typically comprised of four different lipids (fats) that envelop their therapeutic cargo. While these traditional lipid nanoparticles are effective at transporting and protecting their payload, when infused into a patient, they accumulate in one specific organ: the liver.
“Traditional lipid nanoparticles are remarkably similar to low-density lipoprotein (LDL) in terms of their size and chemical composition,” explained Siegwart. “LDL particles naturally travel to the liver to be broken down, so it makes sense that lipid nanoparticles accumulate there as well.”
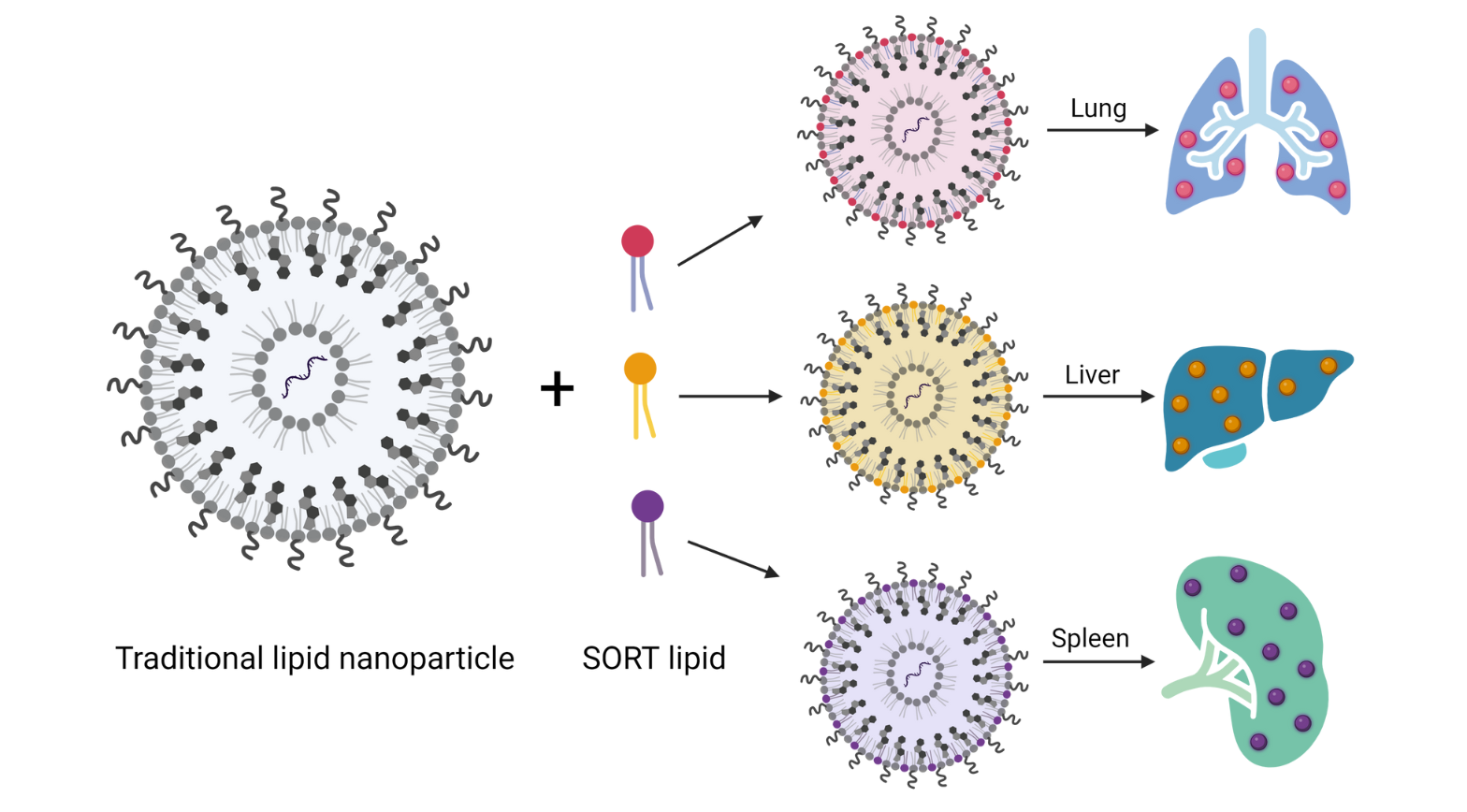
To enable delivery to tissues other than the liver, Siegwart and colleagues previously developed a new class of particle, termed selective organ targeting (SORT) nanoparticles. In addition to the four conventional lipids found in traditional lipid nanoparticles, SORT nanoparticles contain a fifth lipid that directs the particles to a specific organ. This fifth lipid affects the physiochemical properties of the nanoparticle and attracts distinct plasma proteins to its surface, two factors that influence uptake by different types of tissues in the body.
“We knew that we needed to break the rules of traditional lipid nanoparticle formulations to target tissues other than the liver,” Siegwart said. “Using SORT, we’ve shown that we can direct nanoparticles specifically to the liver, spleen, and lungs of mice. Whether this technique could result in durable, tissue-specific gene editing remained an open question.”
Long-term lung editing
Getting the lipid nanoparticles to the right organ is an important first step. But for effective gene editing, it’s also important to target the right type of cell—specifically, stem cells and progenitor cells, or cells that can become different types of cells.
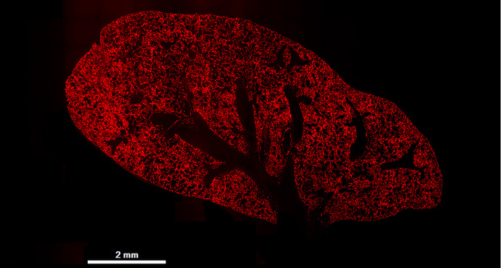
“It’s estimated that the cells in the lungs of rodents turn over and regenerate every few months or so,” said Siegwart. “If you achieve genome correction in mature cells, the effects will be temporary: as soon as the new cells are born, they will no longer have those corrected events, and malfunctioning genes will be produced again.”
To understand if their SORT nanoparticles could achieve durable genome editing in the lungs, Siegwart and colleagues used a genetically engineered mouse that has the capacity to make a red fluorescent protein—but only if its genome is edited in a specific way. “It’s a beautiful model because it allows us to quantify which specific cell types have been edited by the nanoparticles, and we can easily visualize where these nanoparticles are effective,” he said.
The researchers administered SORT nanoparticles filled with gene-editing molecules to mice and then evaluated lung tissues at ten different time points, ranging from two days to 22 months after the injections. They found that the red fluorescence was uniformly spread throughout the lungs at every time point. Further, cell-specific analyses revealed that genome editing was achieved in multiple different types of cells, and that the editing was sustained over the course of the study, nearly two years after treatment with the nanoparticles.
“When we tracked the animals over time, we found that these genome editing events were completely persistent, and almost two years later, the animals were equally edited as they were on the second day,” Siegwart said. “This indicates that the nanoparticles can successfully edit stem and progenitor cell populations that then differentiate over time into healthy, edited cells.”
Evaluation in cystic fibrosis models
With a method to achieve long-term gene editing in hand, Siegwart and colleagues turned their attention to a genetic lung disease: cystic fibrosis.
Cystic fibrosis is caused by mutations in a chloride pump, a protein that can regulate the concentration of salt inside and outside of the cell, Siegwart explained. “When this protein malfunctions, there’s an improper salt balance, leading to thick and sticky mucus building up in the lungs. This can lead to a whole host of different respiratory issues, infections, and eventually persistent lung damage that may lead to the necessity of having a lung transplant.”
Roughly 90 percent of patients with cystic fibrosis can be treated with a breakthrough medicine that helps this chloride pump function more efficiently. However, some patients have mutations in their genome that lead to a truncated, non-functional form of this protein—or the protein is not made at all. These patients currently have no approved treatment options.
In this backdrop, Siegwart and colleagues focused on one of these “undruggable” cystic fibrosis mutations. They filled their lung SORT nanoparticles with a gene editor that corrects the mutation, turning the truncated chloride pump into the normal version of the protein. Then they evaluated how well their system performed in cystic fibrosis models.
In experiments using patient-derived cystic fibrosis lung cells, the researchers found that lung SORT could correct the faulty gene, effectively restoring the function of the chloride pump by more than 50%. What’s more, in a mouse model carrying this undruggable human cystic fibrosis mutation, the researchers found that lung SORT could achieve gene correction in nearly 50% of lung stem cells.
“Our early results suggest that this technique could someday correct dysfunctional proteins in the lungs, which would absolutely be transformative in the daily lives of cystic fibrosis patients,” Siegwart said.
Jermont Chen, Ph.D., a program director in the Division of Discovery Science and Technology at NIBIB, agreed: “Through clever modifications of standard lipid nanoparticles, this team has laid the groundwork for an in vivo gene editing platform in the lungs, which could potentially be translated to other tissues down the line. While future work in relevant animal models will be required before this technique can be evaluated in humans, the method described here has the potential to lead to long-lasting treatments for patients with genetic conditions.”
Note: Siegwart is a co-founder of the company ReCode Therapeutics, a partner in this study.
This study was supported in part by a grant from NIBIB (R01EB025192).
This science highlight describes a basic research finding. Basic research increases our understanding of human behavior and biology, which is foundational to advancing new and better ways to prevent, diagnose, and treat disease. Science is an unpredictable and incremental process—each research advance builds on past discoveries, often in unexpected ways. Most clinical advances would not be possible without the knowledge of fundamental basic research.
Study reference: Yehui Sun et al., In vivo editing of lung stem cells for durable gene correction in mice. Science 384,1196-1202(2024). DOI:10.1126/science.adk9428