NIBIB-funded researchers at the University of Minnesota (UMN) created a new, dynamic 3D bioprinted tumor model in a laboratory dish to screen anticancer drugs and study the spread of cancer and primary site tumor growth. A major pitfall in translating drugs from the lab bench to a viable treatment is delineating the differences that occur inside a 2D petri dish versus the human body, which is inherently 3D. Often, what is observed in a plastic petri dish fails to accurately replicate the conditions and outcomes surrounding tumor growth in a person.
“A 3D system is more effective than 2D at modeling events in the body and informing the drug discovery process. The research community has been moving towards 3D models because they are simply better predictors of what will work in the body,” said Seila Selimovic, Ph.D., director of the NIBIB program in Tissue Engineering.
Fanben Meng, Ph.D., lead researcher in the College of Science and Engineering at UMN used a custom-built 3D bioprinter to inject capsules of proteins into a soft hydrogel. The researchers designed the hydrogel to mimic the composition and stiffness of physiological structures to allow cells to behave in a similar manner outside of the body.
The 3D bioprinting technology used for this project originated in the UMN lab of Michael C. McAlpine, Ph.D., the Benjamin Mayhugh Associate Professor of Mechanical Engineering. He previously showed that 3D printed capsules could release their contents ‘on demand’ with control over where, when, and in which targeted tissues.
The success of this first project led McAlpine and his collaborators to tackle another project, this time by combining these 3D printed biochemical capsules with 3D bioprinted tumor cells. Co-senior author Angela Panoskaltsis-Mortari, Ph.D., the Vice Chair for Research and Professor in the Department of Pediatrics at UMN, said, “Blood vessels help cancer cells migrate to other sites in the body, a process called metastasis, which complicates treatment strategies. I think our model has the key components to push the boundaries of in vitro cancer research.”
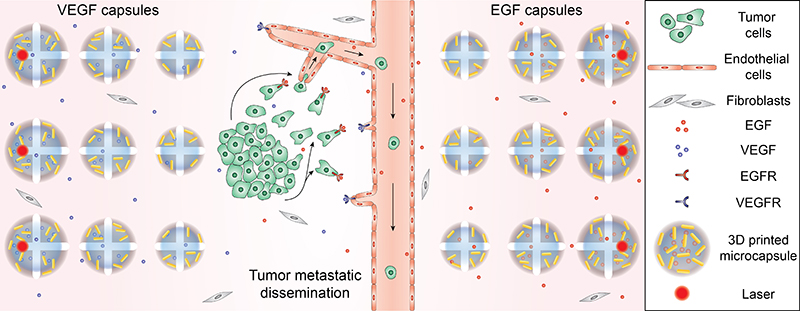
Three-dimensional bioprinting technology was used to precisely place melanoma or lung cancer cells, normal cells, and blood vessel-like structures in the laboratory dish based on their normal functions. Additionally, the hydrogels contain cores that are packed with chemicals to guide cancer cell migration or blood vessel growth, plus an encapsulating outer shell comprised of gold nanorods. The gold nanorods are activated by a laser that heats up the nanorod causing it to rupture the hydrogel core and release its contents. This creates a chemical gradient that guides targeted cell growth. Fluorescence imaging allows researchers to visualize these cancer cells migrating through the constructed blood vessels upon release.
“All these features provide us with the ability to have 4D control – which is control over both space and time,” emphasized McAlpine. “The cells and capsules are precisely printed in biologically relevant sites and the chemical depots propel movement upon a triggered release. This is a dynamic 3D tissue engineering system giving the user control over the diffusion process at some later point after the printing process.” In 2D drug testing systems, cells lie flat on a surface, completely exposed and vulnerable to an anticancer drug on one side and protected by the dish on the other. In this new model, the drug diffuses through the 3D system before it reaches its intended target, the cancer cells.
Results from the UMN study, published in Advanced Materials, found that in the 3D bioprinted model, it takes drugs more time to kill fewer cells than previous studies have shown, but the results likely offer a more accurate representation of the body’s processes than 2D drug screening platforms. The published study also showed that immunotoxins can effectively kill cancer cells using this model as a proof-of-concept.
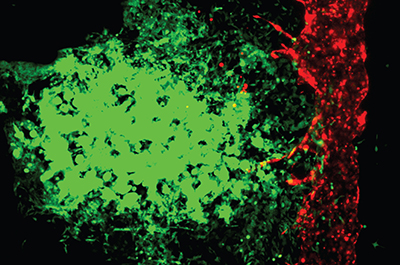
Panoskaltsis-Mortari elaborated, “Other 3D models are not perfusable in the same way. We’ve created a system where we can collect the cells that have self-selected to enter the blood vessel and become metastatic.” Researchers believe collecting these cells in this model may help them understand more about how cancer spreads and how to formulate better treatments.
In discussing manufacturing potential, McAlpine says it is a scalable process because only one bioprinter is needed to print multiple cell types, as well as the hydrogel, the capsules with biochemical factors, and the gold nanorods. Panoskaltsis-Mortari added, “Since the 3D models are bioprinted it is a highly robust, reproducible process and can be used to quickly conduct thousands of tests.”
“As a program director, I want to support research projects that continue to evolve. The evolution of the 3D bioprinting technology from the McAlpine lab is a prime example of how many NIBIB-funded technologies have numerous applications,” said Selimovic enthusiastically. In the future, Panoskaltsis-Mortari and McAlpine plan on developing other models beyond melanoma and lung cancer. Additionally, they will begin to incorporate components of the immune system into their 3D models.
The work was supported by grants from NIBIB (R21EB022830 and DP2EB020537), a seed grant from University of Minnesota Institute for Engineering in Medicine, and a Pilot Project award from the University of Minnesota Prostate and Urologic Cancer Translational Workgroup.
3D bioprinted in vitro metastatic models via reconstruction of tumor microenvironments. Fanben Meng, Carolyn M. Meyer, Daeha Joung, Daniel A. Vallera, Michael C. McAlpine, and Angela Panoskaltsis-Mortari. Advanced Materials. 31, 1806899 2019.